(1) Evaporation : Vaporisation occurring from the free surface of a liquid is called evaporation. Evaporation is the escape of molecules from the surface of a liquid. This process takes place at all temperatures and increases with the increase of temperature. Evaporation leads to cooling because the faster molecules escape and, therefore, the average kinetic energy of the molecules of the liquid (and hence the temperature) decreases.
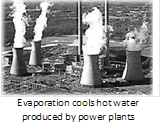
(2) Melting (or fusion)/freezing (or solidification) : The phase change of solid to liquid is called melting or fusion. The reverse phenomenon is called freezing or solidification.
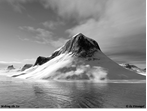
When pressure is applied on ice, it melts. As soon as the pressure is removed, it freezes again. This phenomenon is called regelation.
(3) Vaporisation/liquefication (condensation) : The phase change from liquid to vapour is called vaporisation. The reverse transition is called liquefication or condensation.
(4) Sublimation : Sublimation is the conversion of a solid directly into vapours. Sublimation takes place when boiling point is less than the melting point. A block of ice sublimates into vapours on the surface of moon because of very very low pressure on its surface. Heat required to change unit mass of solid directly into vapours at a given temperature is called heat of sublimation at that temperature.
(5) Hoar frost : Direct conversion of vapours into solid is called hoar frost. This process is just reverse of the process of sublimation, e.g., formation of snow by freezing of clouds.
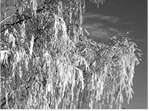
(6) Vapour pressure : When the space above a liquid is closed, it soon becomes saturated with vapour and a dynamic equilibrium is established. The pressure exerted by this vapour is called Saturated Vapour Pressure (S.V.P.) whose value depends only on the temperature ? it is independent of any external pressure. If the volume of the space is reduced, some vapour liquefies, but the pressure is unchanged.
A saturated vapour does not obey the gas law whereas the unsaturated vapour obeys them fairly well. However, a vapour differs from a gas in that the former can be liquefied by pressure alone, whereas the latter cannot be liquefied unless it is first cooled.
(7) Boiling : As the temperature of a liquid is increased, the rate of evaporation also increases. A stage is reached when bubbles of vapour start forming in the body of the liquid which rise to the surface and escape. A liquid boils at a temperature at which the S.V.P. is equal to the external pressure.
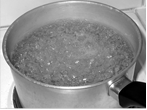
It is a fast process. The boiling point changes on mixing impurities.
(8) Dew point : It is that temperature at which the mass of water vapour present in a given volume of air is just sufficient to saturate it, i.e. the temperature at which the actual vapour pressure becomes equal to the saturated vapuor pressure.
(9) Humidity : Atmospheric air always contains some
more...