(1) Stimulated absorption : Consider an atom which has an allowed state at energy \[{{E}_{1}}\] and another allowed state at a higher energy \[{{E}_{2}}\]. Suppose the atom is in the lower energy state \[{{E}_{1}}\]. If a photon of light having energy \[{{E}_{2}}-{{E}_{1}}\] is incident on this atom, the atom may absorb the photon and jump to the higher energy state \[{{E}_{2}}\]. This process is called stimulated absorption of light photon. The incident photon has stimulated the atom to absorb the energy.
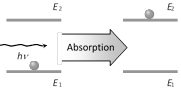
(2) Spontaneous emission : If an atom is present in the higher energy state, it tends to return to the lower energy state within a time of \[{{10}^{-8}}\] sec by emitting a photon of energy \[hv={{E}_{2}}-{{E}_{1}}\]. We call this process spontaneous emission. Spontaneous because the event was not triggered by any outside influence.
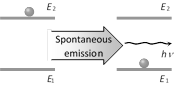
(3) Stimulated emission : Suppose a photon of energy \[hv={{E}_{2}}-{{E}_{1}}\] interacts with an atom that is already in the excited state \[{{E}_{2}}\].
The incident photon may stimulate the atom to emit a photon, the energy, phase, and direction of travel of this second photon are exactly the same as those of the incident photon. That is the quantum state of the stimulated photon is identical to that of the incident photon. This process is called stimulated emission.
If these two photons then interact with two more excited state atoms, two more photons are produced, and soon. Therefore, the stimulation process leads to photon amplification.
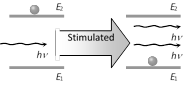
(4) Population inversion : Usually the number of atoms in the lower energy state is more than the number of atoms in the excited state. To emit photons which are coherent (i.e. in phase), the number of atoms in the higher state must be greater than the number of atoms in the lower energy state. In other words, population of atoms in the higher energy state must be larger than the population of atoms in the lower energy state. The process of making the population of atoms in the higher energy state more than that of lower energy state is known as population inversion.
The method used to invert the population of atoms is known as pumping.
(5) Metastable states : A metastable state is one, which has a mean life time of the order of \[{{10}^{-3}}\,s\] or more i.e. much larger than \[{{10}^{-8}}\,s\], the life time of a higher energy state. Some atomic systems, such as chromium, neon, etc possess metastable states. The atom of such an atomic system, when in higher energy state, does not come down to lower energy state directly. It first returns to metastable state and then after a finite lapse of time of the order of \[{{10}^{-3}}\,s\], returns to the lower energy state. Since such atom stays in metastable state for a sufficiently long time, the population inversion can sustain in such atomic system.
A system in which population inversion is achieved
more...