A bar magnet consist of two equal and opposite magnetic pole separated by a small distance. Poles are not exactly at the ends. The shortest distance between two poles is called effective length \[({{L}_{e}})\] and is less than its geometric length \[({{L}_{g}})\]. For bar magnet \[{{L}_{e}}=2l\] and \[{{L}_{e}}=(5/6)\,{{L}_{g}}\]. For semi circular magnet \[{{L}_{g}}=\pi R\] and \[{{L}_{e}}=2R\]
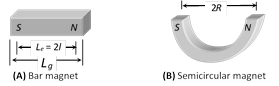
(1) Directive properties : When a magnet suspended freely it stays in the earth's N-S direction (in magnetic meridian).

(2) Monopole concept : If a magnet is Broken into number of pieces, each piece becomes a magnet. This in turn implies that monopoles do not exist. (i.e., ultimate individual unit of magnetism in any magnet is called dipole).
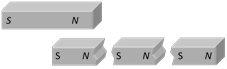
(3) For two rods as shown, if both the rods attract in figure (A) and doesn't attract in figure (B) then, Q is a magnetic and P is simple iron rod. Repulsion is sure test of magnetism.
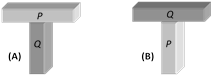
(4) Pole strength (m) : The strength of a magnetic pole to attract magnetic materials towards itself is known as pole strength.
(i) It is a scalar quantity.
(ii) Pole strength of N and S pole of a magnet is conventionally represented by +m and \[-m\] respectively.
(iii) It's SI unit is amp \[\times \] m or N/Tesla and dimensions are [LA].
(iv) Pole strength of the magnet depends on the nature of material of magnet and area of cross section. It doesn't depends upon length.

(5) Magnetic moment or magnetic dipole moment \[(\overrightarrow{M})\] : It represents the strength of magnet. Mathematically it is defined as the product of the strength of either pole and effective length. i.e. \[\overrightarrow{M}=m(2\overrightarrow{\,l\,})\]
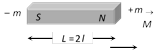
(i) It is a vector quantity directed from south to north.
(ii) It's S.I. unit \[amp\times {{m}^{2}}\] or N-m / Tesla and dimensions \[[A{{L}^{2}}]\]
(6) Cutting of a rectangular bar magnet : Suppose we have a rectangular bar magnet having length, breadth and mass are L, b and w respectively if it is cut in n equal parts along the length as well as perpendicular to the length simultaneously as shown in the figure then
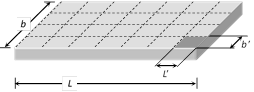
Length of each part \[L'=\frac{L}{\sqrt{n}}\], breadth of each part \[b'=\frac{b}{\sqrt{n}}\] , Mass of each part \[w'=\frac{w}{n}\], pole strength of each part \[m'=\frac{m}{\sqrt{n}}\], Magnetic moment of each part \[M'=m'L'=\frac{m}{\sqrt{n}}\times \frac{L}{\sqrt{n}}=\frac{M}{n}\]
If initially moment of inertia of bar magnet about the axes passing from centre and perpendicular to it's length is \[I=w\,\left( \frac{{{L}^{2}}+{{b}^{2}}}{12} \right)\] then moment of inertia of each part \[I'=\frac{I}{{{n}^{2}}}\]
(7) Cutting of a thin bar magnet : For thin magnet \[b=0\] so \[L'=\frac{L}{n}\], \[w'=\frac{w}{n}\], \[m'=\frac{m}{n}\], \[I'=\frac{I}{{{n}^{3}}}\]